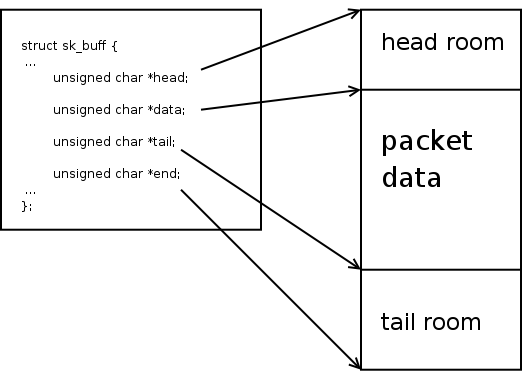
Introduction
While working on a current research project, I started thinking about socket API calls such as send()
and recv()
and their primitive form. I decided to investigate how the Linux kernel processes network data when the send()
method is called. In this post, I will describe the control flow for a TCP packet.
This post assumes that the reader has a strong understanding of TCP/IP networking.
System Call send()
To begin, the send
system call is registered, and simply calls the function __send_sendto()
:
send() syscall registration
SYSCALL_DEFINE4(send, int, fd, void __user *, buff, size_t, len,
unsigned int, flags)
{
return __sys_sendto(fd, buff, len, flags, NULL, 0);
}
__sys_sendto( … ) call
int __sys_sendto(int fd, void __user *buff, size_t len, unsigned int flags,
struct sockaddr __user *addr, int addr_len)
{
struct socket *sock;
struct sockaddr_storage address;
int err;
struct msghdr msg;
int fput_needed;
err = import_ubuf(ITER_SOURCE, buff, len, &msg.msg_iter);
if (unlikely(err))
return err;
sock = sockfd_lookup_light(fd, &err, &fput_needed);
if (!sock)
goto out;
msg.msg_name = NULL;
msg.msg_control = NULL;
msg.msg_controllen = 0;
msg.msg_namelen = 0;
msg.msg_ubuf = NULL;
if (addr) {
err = move_addr_to_kernel(addr, addr_len, &address);
if (err < 0)
goto out_put;
msg.msg_name = (struct sockaddr *)&address;
msg.msg_namelen = addr_len;
}
flags &= ~MSG_INTERNAL_SENDMSG_FLAGS;
if (sock->file->f_flags & O_NONBLOCK)
flags |= MSG_DONTWAIT;
msg.msg_flags = flags;
err = __sock_sendmsg(sock, &msg);
out_put:
fput_light(sock->file, fput_needed);
out:
return err;
}
__sock_sendmsg() -> sock_sendmsg_noec()
A brief note about INDIRECT_CALL_INET, indirect calls, in this context, they are used as an alternative to if/else conditionals to determine the function that the function pointer points to. Presumably, this method is preferred to avoid using branch instructions (and potential misprediction) and the overhead. The sock->ops->sendmsg is a function pointer; for INET messages, the inet_sendmsg routine will be selected.
static inline int sock_sendmsg_nosec(struct socket *sock, struct msghdr *msg)
{
int ret = INDIRECT_CALL_INET(READ_ONCE(sock->ops)->sendmsg, inet6_sendmsg,
inet_sendmsg, sock, msg,
msg_data_left(msg));
BUG_ON(ret == -EIOCBQUEUED);
if (trace_sock_send_length_enabled())
call_trace_sock_send_length(sock->sk, ret, 0);
return ret;
}
The function pointer is defined in linux/include/linux/net.h and takes the following arguments.
int (*sendmsg) (struct socket *sock, struct msghdr *m,
size_t total_len);
If you’d like to understand how the indirect call is used, please visit the indirect_call_wrapper.h header.
inet_sendmsg() -> Indirect_call_2(tcp or udp)
The inet_sendmsg routine uses another indirect call; the function pointer will point to the TCP or UDP sendmsg implementation. The TCP sendmsg routine calls tcp_sendmsg_lock(). The socket is locked
before data is transmitted.
int inet_sendmsg(struct socket *sock, struct msghdr *msg, size_t size)
{
struct sock *sk = sock->sk;
if (unlikely(inet_send_prepare(sk)))
return -EAGAIN;
return INDIRECT_CALL_2(sk->sk_prot->sendmsg, tcp_sendmsg, udp_sendmsg,
sk, msg, size);
}
int tcp_sendmsg(struct sock *sk, struct msghdr *msg, size_t size)
{
int ret;
lock_sock(sk);
ret = tcp_sendmsg_locked(sk, msg, size);
release_sock(sk);
return ret;
}
Moving TCP to IP Layer
The implementation of tcp_sendmsg_locked
includes a call to __tcp_push_pending_frames, and this is where things get interesting. Pushing page frames involves invoking tcp_write_xmit, which traverses the write queue that belongs to the socket and clones the packet.
[!Note] You’ll notice several function calls that are wrapped with likely() or unlikley(). This is a classification used with ELF binaries to improve locality. See my note about this in the post on Linkers in the section
GNU Linker Script Explanation
.
tcp_write_xmit() -> tcp_transmit_skb()
Next, the function tcp_transmit_skb is called, which builds the TCP header. Once complete, the queue_xmit
function pointer is called which invokes IPv4 or IPv6 processing. The respecitve INET routine will encapsulate the buffered TCP (or UDP) data.
static int __tcp_transmit_skb(struct sock *sk, struct sk_buff *skb,
int clone_it, gfp_t gfp_mask, u32 rcv_nxt)
{
////////////////////////////
.... ( LINES OMITTED ) ...
///////////////////////////
err = INDIRECT_CALL_INET(icsk->icsk_af_ops->queue_xmit,
inet6_csk_xmit, ip_queue_xmit,
sk, skb, &inet->cork.fl);
if (unlikely(err > 0)) {
tcp_enter_cwr(sk);
err = net_xmit_eval(err);
}
if (!err && oskb) {
tcp_update_skb_after_send(sk, oskb, prior_wstamp);
tcp_rate_skb_sent(sk, oskb);
}
return err;
}
A similar process follows for each of the lower layers.
Understanding the SKB Data structure
It’s important that you understand the SKB data structure, which is used to represent network packets. There are several sources online that discuss SKB; however, I found the official Linux kernel documentation to be the most helpful.
Why is this important
Current research in High-Performance Computing (which typically defines the initiaives of network developers) is exploring alternative ways to efficiently transmit large amounts of data. Zero-copy networking is a popular strategy that involves bypassing the kernel networking stack (every I explainned above), to allow data movement from user-space buffers directly to the NIC. Furthermore, cloud platforms like Azure, have adopted the use of RDMA to support buffer-to-buffer transmission.
Understanding how current implementations of TCP/IP work is crucial for discovering improvements. I was recently introduced to DPDK (Data plane Development Kit); a frameware for implementing zero-copy networking and an abstraction layer in the networking model. Other interesting projects include Named Data Networking, which is one of the five initiatives sponsered by the National Science Foundation for future architecutre of the internet.